Biochemical Calculations I H Segel Wiley 1976 Pdf Creator
Abstract Nitrogenase catalyzes the MgATP-dependent reduction of dinitrogen gas to ammonia. In addition to the physiological substrate, nitrogenase catalyzes reduction of a variety of other multiply bonded substrates, such as acetylene, nitrous oxide, and azide. Although carbon monoxide (CO) is not reduced by nitrogenase, it is a potent inhibitor of all nitrogenase catalyzed substrate reductions except proton reduction. Here, we present kinetic parameters for an altered Azotobacter vinelandii MoFe protein for which the αGly 69residue was substituted by serine (Christiansen, J., Cash, V. L., Seefeldt, L. C., and Dean, D.
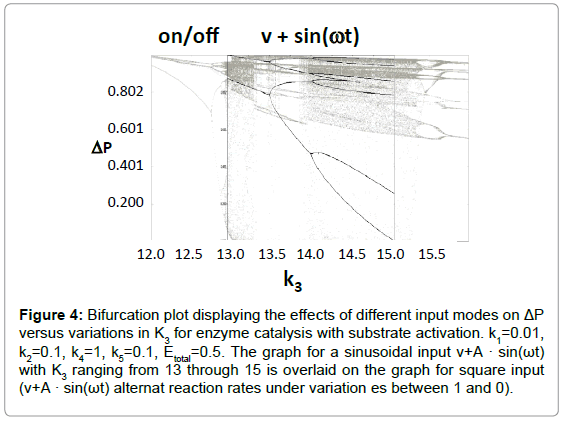
For the wild type enzyme, CO and acetylene are both noncompetitive inhibitors of dinitrogen reduction. However, for the αSer 69 MoFe protein both CO and acetylene have become competitive inhibitors of dinitrogen reduction. Ace Combat 5 Joystick Drivers. CO is also converted from a noncompetitive inhibitor to a competitive inhibitor of acetylene, nitrous oxide, and azide reduction. These results are interpreted in terms of a two-site model.
Conflict Resolution And Prevention John Burton Pdf Creator read more. Codejock Crack Cocaine. Biochemical Calculations I H Segel Wiley 1976 Pdf To Jpg.
Site 1 is a high affinity acetylene-binding site to which CO also binds, but dinitrogen, azide, and nitrous oxide do not bind. This site is the one primarily accessed during typical acetylene reduction assays.
Site 2 is a low affinity acetylene-binding site to which CO, dinitrogen, azide, and nitrous oxide also bind. Site 1 and site 2 are proposed to be located in close proximity within a specific 4Fe-4S face of FeMo cofactor. The site of substrate binding and reduction within the Mo-dependent nitrogenase is provided by a complex metallocluster located within the α-subunit of the MoFe protein (,). This metallocluster, called FeMo cofactor, contains a metal-sulfur core constructed from 4Fe-3S and 3Fe-3S-Mo subfragments that are geometrically analogous to pieces derived from cuboidal clusters () (Fig. An organic constituent, homocitrate, is coordinated to the Mo atom through its 2-hydroxy and 2-carboxyl groups. Opposite ends of the FeMo cofactor are covalently attached to the MoFe protein α-subunit through αCys 275, coordinated to an Fe atom at one end of the molecule, and αHis 442, which is coordinated to the antipodal Mo atom. The FeMo cofactor also interacts with the MoFe protein through a number of direct and water-bridged hydrogen bonds.
Where and how dinitrogen interacts with the metal-sulfur surface of FeMo cofactor during catalysis and the specific contribution of the polypeptide to that process has been the subject of much speculation and debate (), but there is little substantive information available concerning these issues. Figure 1 a, ball-and-stick representation of the FeMo cofactor showing the Fe-S framework, peptide ligands αHis 442 and αCys 275 as well as the homocitrate moiety. Iron atoms are shown in green, sulfur in yellow, and molybdenum is shown magenta.
B, view looking down the long axis of the FeMo cofactor, showing the residues approaching the 4Fe-4S “active face.” c, stereoview looking at the FeMo cofactor shown in b, from the top, with the αHis 195residue removed. The dotted lines indicate possible hydrogen bond interactions between the backbone of residues Gly 69-Val 70 and the guanidino group of Arg 96. Figures were generated using Raster3D (, ) and MOLSCRIPT (). In addition to an ability to reduce its natural substrate, nitrogenase also catalyzes reduction of a variety of other multiply bonded substrates, including acetylene, azide, and nitrous oxide (). In the absence of dinitrogen, or other substrates, nitrogenase catalyzes the reduction of protons to yield hydrogen gas.
Carbon monoxide (CO) is not a nitrogenase substrate but is a powerful inhibitor of all nitrogenase catalyzed substrate reductions except proton reduction. In the presence of CO, electron flux through nitrogenase is diverted to proton reduction whether or not any other substrate is available. The kinetic features of nitrogenase as well as the nature of the interaction among the various nitrogenase substrates and inhibitors are complex. This complexity has been attributed to multiple substrate and inhibitor binding sites within the enzyme (, ) and the fact that the enzyme accesses a range of redox states during turnover ().
These features have hampered the use of inhibitors and alternative substrates to identify and characterize the physiologically relevant active site. One approach toward solving this problem is to isolate an altered form of nitrogenase for which the physiologically irrelevant inhibitor and substrate binding site(s) have been eliminated without causing a significant alteration in the ability of the enzyme to reduce dinitrogen. Rdb Tetho Mera Door Jaan Nu Free Mp3 Download. Toward this end we recently described a genetic selection for the isolation of a MoFe protein that is severely altered in its ability to reduce the alternative substrate acetylene but is only modestly affected in its ability to reduce dinitrogen (). Here we report on the ability of this altered MoFe protein, which has the αGly 69 residue substituted by serine, to reduce the alternative substrates azide and nitrous oxide, as well as on the susceptibility of the reduction of these and other substrates to inhibition by CO. Protein Isolation and Activity Assays MoFe proteins were isolated from A.
Vinelandii strains designated DJ995 (αGly 69, wild type) () or DJ1262 (αSer 69) (). Methods for strain construction, large scale growth, protein purification, and handling were reported previously (, ). All manipulations of isolated nitrogenase proteins were done anaerobically using a schlenk apparatus (). Assays consisted of a 1-ml final volume containing the nitrogenase component proteins, 25 m m HEPES buffer, pH 7.4, Na 2S 2O 4 and a MgATP regenerating system (). Assay samples that had gaseous substrates added were brought to atmospheric pressure prior to the start of the assay. The reaction, performed at 30 °C, was initiated by the addition of Fe protein and terminated by addition of 0.25 ml of 0.4 mEDTA, pH 7.5. In solution, azide is present as both N 3 − and HN 3, and “azide” will therefore refer to these two species collectively.
Azide was prepared from a 0.1 m stock solution of NaN 3 prepared in 25 m m HEPES buffer, pH 7.4. Gaseous dinitrogen, nitrous oxide, and CO were taken directly from commercially prepared high purity cylinders, and acetylene was prepared fresh by the reaction of calcium carbide with water. For the measurement of H 2, a Shimadzu GC-14 gas chromatograph equipped with a molecular sieve 5A column and a thermal conductivity detector was used. To measure ethylene and methane, a Hewlett-Packard 5890A gas chromatograph equipped with an Al 2O 3 capillary column and flame ionization detector was used. NH 3 was quantified with the colorimetric indophenol method (, ), and hydrazine (for detecting azide reduction) was measured using the reaction with p-dimethylaminobenzaldeyhde (). Treatment of Kinetic Data Lineweaver-Burk plots were used to initially determine the pattern of inhibition. All data used was the average of at least four independent determinations.
Data for the substrate alone and with at least two inhibitor concentrations were then plotted as velocity versus substrate concentration and fit to the following hyperbolic equation (), where v is the measured enzyme velocity, [ S] is the substrate concentration, and the variable parameters V max ′ and K m ′ denote the apparent maximum velocity and Michaelis constant (when the inhibitor concentration is zero, V max ′ = V max and K m ′ = K m). Values from the resulting linear fits could then be used to generate linear replots of the data from which values for K is (replotted as K m/ V max versusinhibitor concentration for both competitive and noncompetitive inhibition) and K ii (replotted as 1/ V max versus inhibitor concentration for noncompetitive inhibition) could be derived. RESULTS AND DISCUSSION The K m values determined for dinitrogen and the alternative substrates acetylene, azide, and nitrous oxide are shown in Table for both the wild type (αGly 69) and αSer 69 MoFe proteins. The results show that the dinitrogen, N 2O, and azide K m values for both MoFe proteins are similar. However, the small increase in the dinitrogen K mfor the αSer 69 MoFe protein and relatively larger increase in K m for N 2O and azide are reproducible. In contrast to these substrates, there is a marked 20-fold increase in the K m for acetylene reduction catalyzed by the αSer 69 MoFe protein when compared with the wild type MoFe protein. Although not a substrate, CO is reported to be a powerful inhibitor of all nitrogenase substrate reduction activities except for proton reduction ().
We are able to reproduce this effect for CO inhibition of dinitrogen, acetylene, azide, and nitrous oxide reduction. Table shows the K is and K ii values we obtain for the wild type enzyme. Representative data used to obtain these values are shown in Lineweaver-Burk plots presented in Fig. In the case of the αSer 69 MoFe protein, CO is converted from a noncompetitive to a competitive inhibitor of all four substrate reductions (Table and Fig. Comparison of the K is values with respect to CO obtained for the wild type MoFe protein and the αSer 69 MoFe protein shows that they are all quite similar.
This indicates that this aspect of the interaction of CO with the MoFe protein is unchanged between the wild type and αSer 69 MoFe proteins. Figure 2 Lineweaver-Burk plots showing the change from a noncompetitive inhibition pattern to a competitive inhibition pattern for CO inhibition of acetylene in the wild type ( a) and αSer 69 MoFe proteins ( b). The same pattern change observed for CO inhibition of nitrogen reduction is shown for the wild type ( c) and αSer 69 MoFe proteins ( d). For all plots, the enzyme in the absence of inhibitor is indicated by open circles. For enzyme in the presence of 0.0002 atm CO, the data is indicated by filled squares.
For the wild type MoFe protein, acetylene is a noncompetitive inhibitor of dinitrogen, nitrous oxide, and azide reduction (, ). We previously reported that substitution of the αGly 69residue by serine results in an altered MoFe protein for which acetylene is now a competitive inhibitor of dinitrogen reduction (). The αSer 69 substitution also results in a dramatic increase in K m for acetylene reduction. On the basis of these results we concluded that the normal enzyme has two distinct acetylene binding sites. One of these (Site 1) has a high affinity for acetylene binding ( K m ∼0.007 atm) and is functionally separate from the site to which dinitrogen and other mutually competitive substrates, for example azide () and nitrous oxide (), bind.
The other site (Site 2) has a much lower affinity for acetylene binding ( K m ∼0.14 atm) and is functionally equivalent to the site where dinitrogen binds. Thus, the ability of acetylene to access Site 1 appears to have been eliminated or severely altered as a result of the αSer 69substitution, resulting in the sole manifestation of Site 2. Similar to the situation of acetylene inhibition of the reduction of other substrates catalyzed by the αSer 69 MoFe protein, the altered MoFe protein exhibits a competitive rather than noncompetitive pattern of inhibition by CO for the reduction of all triply bonded substrates examined, including acetylene.
Our interpretation of these results is that, like acetylene, there are two different CO binding sites that affect substrate reduction when occupied by CO. One of these corresponds to the same site or an overlapping site as the high affinity acetylene binding site (Site 1) and the other corresponds to the low affinity acetylene binding site (Site 2), which is also the same site to which dinitrogen, azide, and nitrous oxide bind. It should be noted that, because the MoFe protein accesses a manifold of different redox states during turnover, it is possible that Site 1 and Site 2 might not necessarily differ in their physical locations, but could represent different redox states of the enzyme. There is also no direct kinetic evidence to indicate that both CO and acetylene bind to Site 1. However, this assignment is consistent with the observation that a single amino acid substitution within the MoFe protein is able to convert both CO and acetylene from noncompetitive to competitive inhibitors of the reduction of other triply bonded substrates.
Previous EPR and electron nuclear double resonance spectroscopic studies have been interpreted to indicate that CO binds to Fe in the FeMo cofactor (, ). Thus, because CO becomes a competitive inhibitor of substrates for the αSer 69 MoFe protein, it can be inferred that at least the initial substrate binding event is likely to occur at a site involving Fe in the FeMo cofactor. However, this possibility must be considered from the perspective that no direct kinetic relationship has yet been established between individual EPR species generated during nitrogenase turnover in the presence of CO and effects on substrate reduction. In our original analysis of the αSer 69 MoFe protein we proposed that both the high affinity and low affinity acetylene binding sites are functionally distinct but located within the same 4Fe-4S face of the FeMo cofactor that is approached by the αVal 70 residue (). Inspection of the MoFe protein structure reveals that both the αGly 69 and αVal 70 residues are located on a short helix that skirts one face of FeMo cofactor () (Fig. In addition to the approach of the αVal 70 side chain toward one side of a 4Fe-4S face of the FeMo cofactor, the carbonyl groups of both αGly 69 and αVal 70 are within hydrogen bonding distance of a guanidino group provided by the side chain of αArg 96, which also approaches the same 4Fe-4S face.
Thus, it can be reasonably considered that the αSer 69substitution could affect the accessibility of nitrogenase substrates and inhibitors to this 4Fe-4S face as a result of movement of either the αVal 70 side chain or the main chain that includes αGly 69 and αVal 70, which is interacting with αArg 96. In either case, if all catalytically relevant substrate and inhibitor binding sites are located on the same face, alteration of this environment is expected to have some effect on binding events at both sites. Because the original selection of the mutant that harbors the αSer 69 substitution demanded a capacity for near normal physiological dinitrogen reduction, a dramatic effect on dinitrogen reduction catalyzed by the isolated enzyme from this strain was not expected and is not observed. In contrast, no such selection pressure was placed on the ability of the altered MoFe protein to reduce azide and the K m for the reduction this substrate is significantly increased by the αSer 69 substitution. Azide, nitrous oxide, and dinitrogen are all mutually competitive inhibitors of each other for the wild type MoFe protein and almost certainly bind to the same site.
Thus, in addition to the elimination of the catalytically relevant accessibility of acetylene and CO to Site 1 as a result of the αSer 69 substitution, there is also an effect on certain binding events that occur at Site 2, further indicating that both sites are in close proximity. In summary, with respect to CO, acetylene, azide, nitrous oxide, and dinitrogen, there are two catalytically relevant substrate and inhibitor binding sites located within the nitrogenase MoFe protein. Site 1 is able to bind either acetylene or CO but apparently does not bind dinitrogen, azide, or nitrous oxide. Site 2 is able to bind CO, acetylene, azide, nitrous oxide, and the physiological substrate, dinitrogen. This two-site model was derived from analysis of the αSer 69 MoFe protein for which accessibility to Site 1 is eliminated or severely altered, with respect to effects on substrate reduction, and is consistent with the known kinetic parameters of the wild type enzyme (, ) and other altered MoFe proteins that have been described (, ). We also propose that Site 1 and Site 2 are located within the same 4Fe-4S face of FeMo cofactor that is bounded by αVal 70, αArg 96, and αHis 195(Fig.
The important conclusion that can be made from this work is that when the nonphysiologically relevant substrate and inhibitor site is functionally removed, nitrogenase behaves as a classic single site enzyme where substrate and inhibitor interactions are reciprocal. Footnotes • * This work was supported by National Institutes of Health Grant R01-GM59087.The costs of publication of this article were defrayed in part by the payment of page charges. The article must therefore be hereby marked “ advertisement” in accordance with 18 U.S.C. Section 1734 solely to indicate this fact. • ¶ To whom correspondence may be addressed. Tel.: 540-231-5895; Fax: 540-231-7126; E-mail: deandr@vt.edu (for D.
Dean) or Tel.: 435-797-3964; Fax: 435-797-3390; E-mail: seefeldt@cc.usu.edu (for L. • Published, JBC Papers in Press, August 17, 2000, DOI 10.1074/jbc.M004889200 • Received June 6, 2000. • Revision received July 24, 2000. • The American Society for Biochemistry and Molecular Biology, Inc.